Ask Ethan: Can a lumpy Universe explain dark energy?
Our Universe isn't just expanding, the expansion is accelerating. Instead of dark energy, could a "lumpy" Universe be at fault?
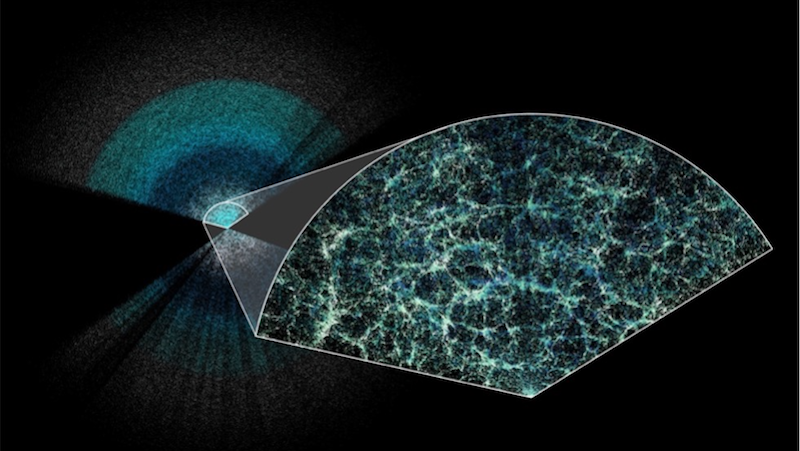
By mapping out the three-dimensional positions of galaxies over a large volume of the Universe, scientists within the DESI collaboration have uncovered some (but not overwhelming) suggestive evidence that the strength of dark energy has weakened (and is weakening) over time. Using the feature of baryon acoustic oscillations (BAOs) may be the method of investigation that finally breaks the Standard Model of cosmology, but the picture with constant dark matter and dark energy still remains strong.
Sign up for the Starts With a Bang newsletter
Travel the universe with Dr. Ethan Siegel as he answers the biggest questions of all
It's now been more than 25 years since astronomers discovered "most of the Universe" in an incredibly surprising way. In terms of energy, the most dominant species in our Universe isn't light, it isn't normal matter, it isn't neutrinos, and it isn't even dark matter. Instead, a mysterious form of energy — dark energy — makes up about ⅔ of the total cosmic energy budget. As revealed by supernovae, baryon acoustic oscillations, the cosmic microwave background, and other key probes of the Universe, dark energy dominates the Universe and has for around ~6 billion years, causing our Universe to not only expand, but for that expansion to accelerate, causing distant galaxies to recede from us with greater and greater speeds as time goes on.
But could all of this be based on an erroneous assumption? Could dark energy not exist at all, and could a lumpy, highly inhomogeneous Universe be the culprit, as one recent study has claimed? That's what many of you, including Dirk Van Tatenhove, Michael Wigner, and Patreon supporter RicL want to know, inquiring things such as:
"Is the timescape model of cosmic expansion a serious threat to the existence of dark energy?
Do you find the timescape hypothesis of cosmic expansion to be credible? If so, would that create a problem with observations that the geometry of the universe is flat on the average?
The model suggests that a clock in the Milky Way would be about 35 percent slower than the same one at an average position in large cosmic voids, meaning billions more years would have passed in voids… 35% sounds an awful lot to me."
Although this might be based on a relatively new study, the idea is quite old. It turns out it runs into colossal problems when confronted not just with supernova data, but with what's already known about the large-scale structure of the Universe. Let's take a look for ourselves.
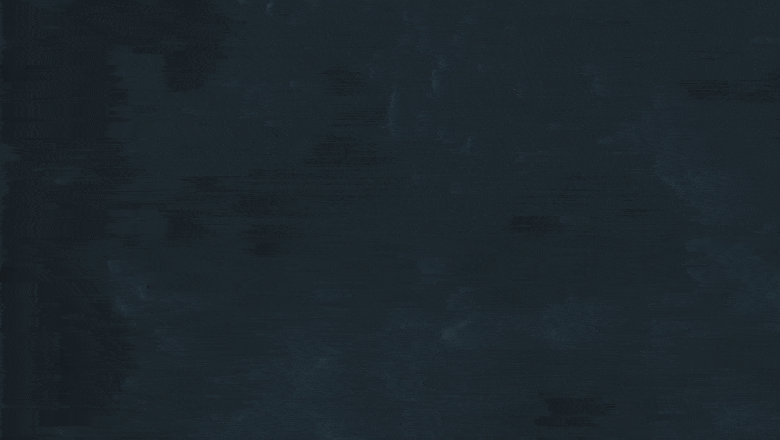
This snippet from a structure-formation simulation, with the expansion of the Universe scaled out, represents billions of years of gravitational growth in a dark matter-rich Universe. Over time, overdense clumps of matter grow richer and more massive, growing into galaxies, groups, and clusters of galaxies, while the less dense regions than average preferentially give up their matter to the denser surrounding areas. The "void" regions between the bound structures continue to expand, but the structures themselves do not.
The first thing you have to understand is that despite how it looks locally, where we have a few objects that are extremely dense compared to the cosmic average (like planets, stars, and galaxies) while most of space is devoid of such objects (interplanetary, interstellar, or intergalactic space) altogether, on large cosmic scales, the Universe is very, very uniform. If you were to take a "dipper" that was the size of a kitchen ladle and "dipped" it into the interior of a star or planet, it would pull out matter with roughly the density of water: 1 gram per cubic centimeter.
But if instead your dipper were enormous, like "10 billion light-years per side" levels of enormous, you'd find that whether you dipped your dipper into:
- an ultra-dense galaxy cluster,
- an ultra-sparse cosmic void,
- or anything in between,
that the average density of what you pulled out would be nearly identical: with about one proton's worth of total energy per cubic meter of space. Even though the difference between underdense and overdense regions (what the pros call "density contrast") is enormous on small cosmic scales, with typical values approaching a factor of ~1030, on the largest of cosmic scales, those density differences are on the order of ~0.01%, or less than 1-part-in-10,000.
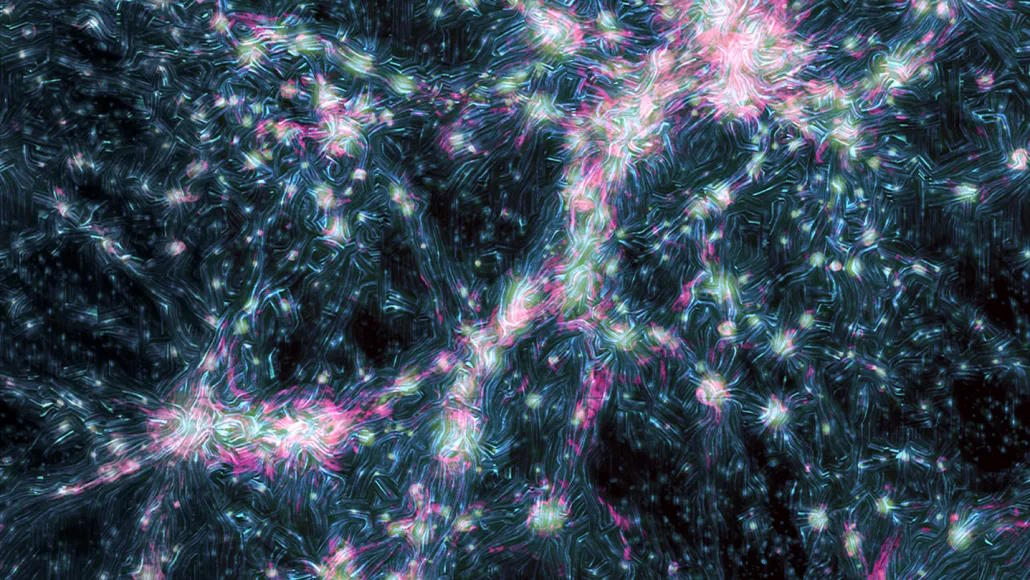
This map comes from a simulation of a portion of the cosmic web, with shock waves (white), magnetic fields (cyan), and radio light (pink) illustrated here. While simulations of the cosmic web can include all sorts of features, the portions of the cosmic web we can observe are limited and biased, and may not serve as a reliable tracer of the underlying matter distribution.
This isn't something that you can measure very well by looking at isolated, random "points" that you might sample in the Universe. You can't look at:
- the brightest, most massive galaxies within the largest galaxy clusters,
- the distribution of gamma-ray bursts,
- the distributions of quasars,
- or the distributions of cataclysms, like individual type Ia supernovae,
and expect that you're going to get a "fair sample" of the Universe. If you insist on using those objects, which are non-representative of the Universe as a whole, you're succumbing to the fallacy of using a biased tracer, which can lead you to believing in the existence of objects, forces, or structures that you would easily see don't exist if you used a better, more comprehensive indicator.
Instead, our best tools for measuring how homogeneous (i.e., uniform) or inhomogeneous (e.g., clumpy or lumpy) the Universe is are twofold.
- We can start here, where we are, and measure how galaxies — large and small, high mass and low mass, luminous and faint, etc. — are distributed across space on all cosmic scales. Using this, we can construct a "mass/density map" of the Universe, not just nearby, but at all points throughout cosmic history.
- Or, we can start at the beginning — with the seed fluctuations produced by inflation — and evolve that forward in time until we reach the cosmic microwave background, and then compare our inhomogeneity map from that time (which we observe) with those theoretical predictions.

Although we can measure the temperature variations all across the sky, on all angular scales, it's the peaks and valleys in the temperature fluctuations that teach us about the ratio of normal matter to dark matter, as well as the length/size of the acoustic scale, where normal matter (but not dark matter) gets "bounced" outward from interactions with radiation. This radiation includes photons, which have a substantial cross-section with particles in the ionized plasma of the early Universe, and neutrinos, which do not.
It should come as no surprise that we've done precisely that with both of these methods. For the second option, we got our best data back in the 2010s from the Planck satellite, and found that the "average density fluctuation" in the early Universe was roughly the same on all scales, large and small, and was at just the 1-part-in-30,000 level. Moreover, we've also accomplished this with the first option, and have found a value that's not only consistent with the other method, but have shown how structure grows and clumps over cosmic time: in perfect agreement on practically all scales with what simulations and theory predicts.
Additionally, many near-future missions (the Vera Rubin observatory, the Nancy Roman telescope, and the SPHEREx mission) will measure cosmic structure more exquisitely than ever, cementing what was first assumed and then observed to be true: that the Universe, on the largest cosmic scales, is incredibly homogeneous and uniform.
It's these facts that justify our longstanding cosmological models: where the Universe is roughly the same everywhere (homogeneous) and in all directions (isotropic), with only small, quantifiable imperfections superimposed atop this uniform background. The Universe was born uniform, then clumped and clustered, and despite all that's transpired, remains relatively uniform on the largest of cosmic scales.

This simulation shows the cosmic web of dark matter and the large-scale structure it forms. Normal matter is present, but is only 1/6th of the total matter. Meanwhile, matter itself only composes about 2/3rds of the entire Universe, with dark energy making up the rest. Although the entropy of our entire Universe is enormous, dominated by supermassive black holes, the entropy density is remarkably small. Even though entropy always increases, in the expanding Universe, entropy density does not.
If we work with a Universe that has these properties, then the only way to "match" what we see with what must exist is to invoke two ingredients that go beyond what's directly known to exist and make up the Universe. In addition to "normal matter" (which includes the familiar protons, neutrons, and electrons), to light (radiation in the form of photons), and to neutrinos (which are part of the Standard Model of known particles), there must also be a large amount of dark matter that outmasses normal matter by a factor of about 5-to-1, and there must also be dark energy, which accounts for about double the energy density of all other forms of mass/energy (including dark matter) combined.
That's our standard model of cosmology, and it has withstood countless challenges throughout the 21st century.
Nevertheless, it's important to keep on challenging the status quo and to explore alternatives, as the idea of attempting to knock down even your most well-established theories and hypotheses is a key component of the enterprise of science. One such alternative to consider that made a lot of noise at the very end of 2024 (and continues now, at the start of 2025) is known as the timescape cosmology, developed by David Wiltshire of New Zealand. In a new paper (and accompanying press release), the claim is that dark energy doesn't need to exist, and that huge differences in energy density between regions of space create a "lumpy" Universe that exhibits wildly different expansion rates and cosmic ages across these various regions of space.
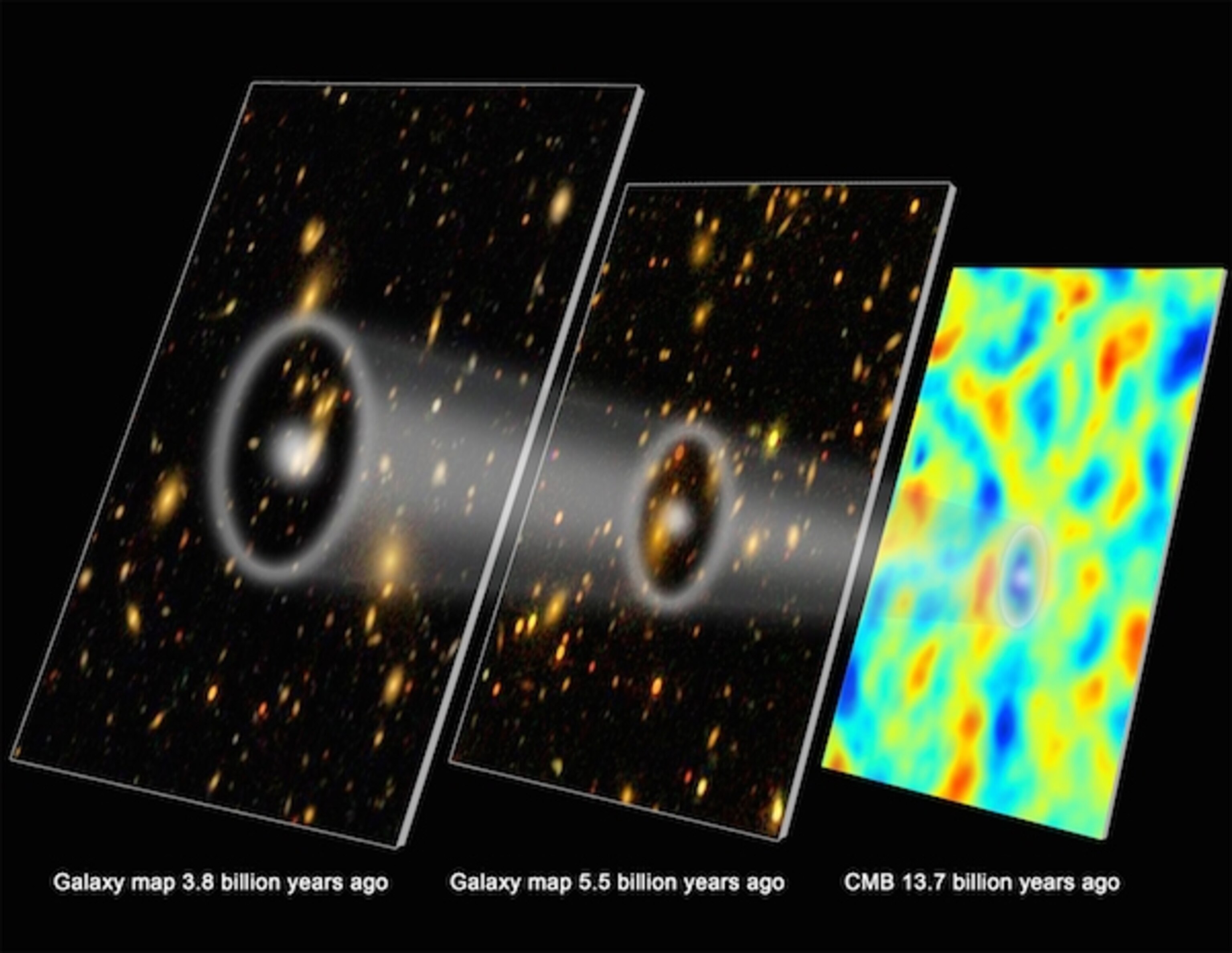
We can look arbitrarily far back in the Universe if our telescopes allow, and the clustering of galaxies should reveal a specific distance scale – the acoustic scale – that should evolve with time in a particular fashion, just as the acoustic "peaks and valleys" in the cosmic microwave background reveal this scale as well. The evolution of this scale, over time, is an early relic that reveals a low expansion rate of ~67 km/s/Mpc, and is consistent from CMB features to BAO features.
If this framework were correct, it would imply many new phenomena.
- The Universe would need to be very inhomogeneous, and the relatively "clumpy" and "empty" regions of space that we find differ not by ~0.01% from one another in density, but by more like ~100% from region-to-region.
- That instead of gravitational time dilation altering the age of one region versus another by up to hundreds or thousands of years compared to the 13.8 billion year age of the overall Universe, those age differences would instead be in the billions of years.
- And that instead of dark energy causing the Universe to accelerate in its expansion, these large-magnitude inhomogeneities alter the local expansion rate severely, creating regions where the expansion rate is either much larger or much smaller than the cosmic average overall.
As many have noted — including astrophysicists I respect such as Brian Koberlein and David Kipping — this falls into the "profound, if true" category.
But is it true?
As the authors argue, if you use type Ia supernovae as the testing ground, you find that both the standard model of cosmology (what we sometimes call ΛCDM, or the dark matter and dark energy-rich but mostly uniform Universe that we know) and the timescape cosmology model work pretty well, and that future studies with many more type Ia supernovae will be able to distinguish between the two.
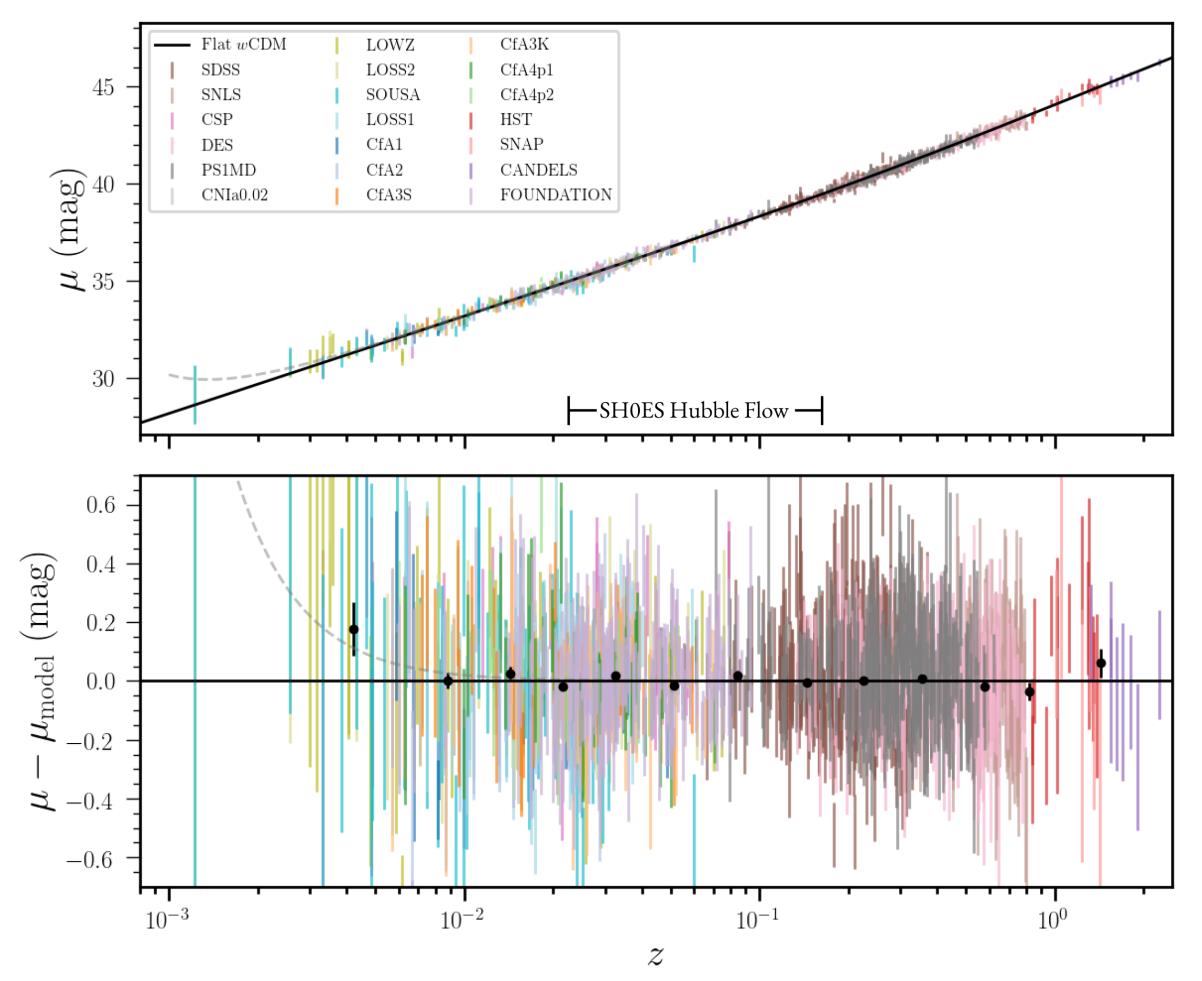
This graph shows the 1550 supernovae that are a part of the Pantheon+ analysis, plotted as a function of magnitude versus redshift. The supernova data, for many decades now (ever since 1998), has pointed toward a Universe that expands in a particular fashion that requires something beyond matter, radiation, and/or spatial curvature: a new form of energy that drives the expansion, known as dark energy. The supernovae all fall along the line that our standard cosmological model predicts, with even the highest-redshift, most far-flung type Ia supernovae adhering to this simple relation. Calibrating the relation without substantial error is of paramount importance.
Unfortunately, however, for the authors and also for anyone buying into their claims, that's not the best testing ground we can muster. The best testing ground for this scenario is to instead look at the structure that's formed in the Universe on all scales, and to test-and-measure how homogeneous vs. inhomogeneous it actually is.
Then, based on that observed level of inhomogeneity, we can simulate a variety of things, including:
- how significantly these cosmic inhomogeneities contribute to the overall energy density,
- what type of effects this "inhomogeneity energy" actually has on the expanding Universe (i.e., whether it behaves as radiation, matter, curvature, dark energy, etc.),
- and how that energy evolves over time, to see whether it can possibly emulate or mimic the effects of dark energy.
Fortunately for all of us, this is not "future work" where the answer is unknown, but work that was done by a large portion of the astrophysics community — including by me, personally — some 20 years ago.
Back in 2005, a team of astrophysicists (Rocky Kolb, Tony Riotto, Sabino Matarrese, and Alessio Notari) suggested a version of this very idea: that dark energy doesn't exist, and that the effects of inhomogeneity energy on the Universe is instead tricking us into seeing an expansion rate that differs from our predictions. Relatively swiftly, the astrophysics community concluded that this could not be the case. Here's how we knew.
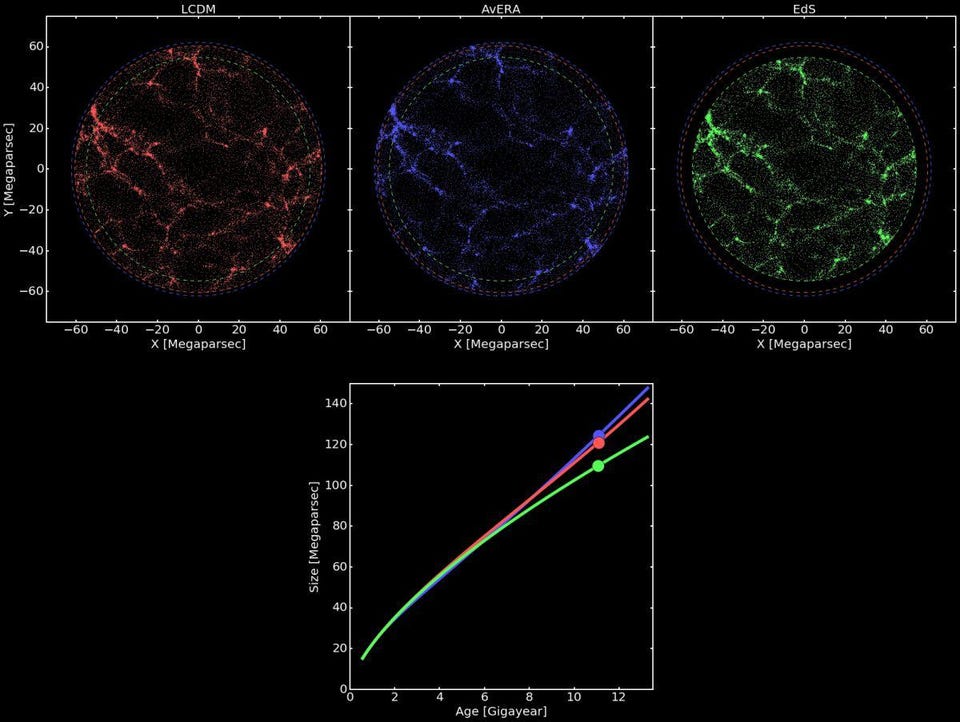
A Universe with dark energy (red), a Universe with large inhomogeneity energy (blue), and a critical, dark-energy-free Universe (green). Note that the blue line behaves differently from dark energy. New ideas should make different, observably testable predictions from the other leading ideas. And ideas which have failed those observational tests should be abandoned once they reach the point of absurdity.
Credit: Gabor Racz et al., 2017
There are both gravitational potential terms (because of gravitational collapse/contraction) and also kinetic terms (because the matter is in motion), and both of those play a role and must be calculated. After performing those calculations — not just with a first-order or second-order approximation, but taking into account fully nonlinear inhomogeneities — a number of lessons emerge.
- It turns out that inhomogeneities, as a function of energy density, always remain small: no greater than about ~0.1% (or 1-part-in-1000) of the total energy density at any time, even many billions of years into the future.
- It also turns out that there's a "key scale" where the greatest contributions arise: on scales of between about a few hundred thousand and around ten million light-years. Both larger and smaller cosmic scales, even including super-horizon scales, contribute less.
- And finally, it turns out that the inhomogeneities never behave as dark energy behaves, and in fact has an equation of state that always contributes further to a decelerating universe, not an accelerating one.
The ending sentences from my 2005 paper, now a full 20 years old, remain tremendously timely, especially with regard to Wiltshire's work and the attention it's been getting. In particular:
"The possibility that a known component of the universe may be responsible for the accelerated expansion remains intriguing. However, we conclude that sub-horizon perturbations are not a viable candidate for explaining the accelerated expansion of the universe."
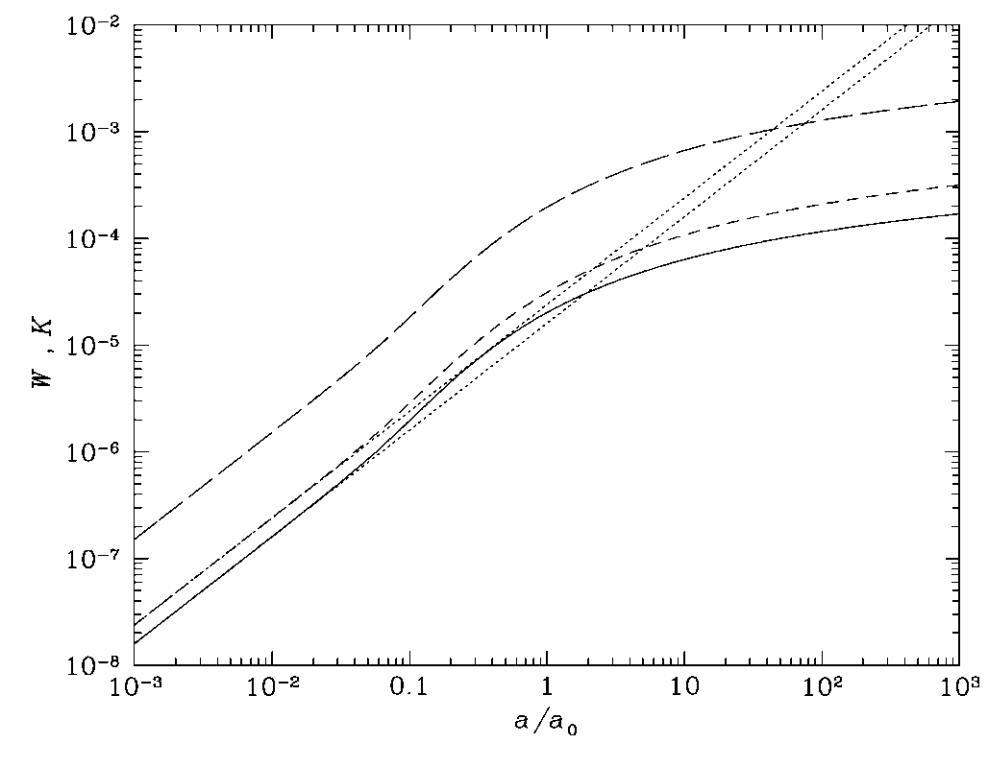
What effects do cosmic inhomogeneities, i.e., the departures from perfect "smoothness," have on the cosmic expansion? The three lines show the contributions to the overall energy density from gravitational potential energy (top), inhomogeneity energy (middle), and kinetic energy (bottom) in the expanding Universe. The y-axis is scaled so that "1" is 100% of the energy density, and the x-axis is scaled so that "1" is today, the past is to the left, and the future is to the right.
There's also something worth pointing out to those of you who aren't experts, but are merely interested onlookers: David Wiltshire, who has been the leading proponent of the timescape cosmology, has been investigating exactly this type of "alternative to dark energy" ever since that idea was first proposed (and debunked) back in 2005. Some example papers include:
- a 2005 paper suggests using type Ia supernovae to suggest there's no dark energy,
- a 2007 paper suggesting that gravitational energy differences lead to the illusion of cosmic acceleration,
- a 2011 paper again suggesting that gravitational energy mimics dark energy and leads to only an apparent acceleration,
- a 2011 paper arguing against a homogeneous universe and in favor of this new "timescape cosmology,"
- a 2017 paper seeking to prove that cosmic acceleration is only an apparent phenomenon from type Ia supernovae,
- and three recent papers arguing the same concept: that dark energy isn't real, and only appears as an apparent effect due to the backreaction of cosmic inhomogeneities.
Despite the fact that we have better type Ia supernovae data today than ever before, this "new research" is just a continuation of a longstanding research program that explores, but in no way proves or validates, an alternative idea to the mainstream. These ideas are important, but the consensus — at least for now — is that our understanding of large-scale structure precludes this from being physically relevant for our own Universe.

This view of about 0.15 square degrees of space reveals many regions with large numbers of galaxies clustered together in clumps and filaments, with large gaps, or voids, separating them. Each point of light is not a galaxy, but a supermassive black hole, revealing just how ubiquitous these cosmic objects are. By estimating the black hole mass function across cosmic time, researchers have a suggestive solution to the "seeds of supermassive black holes" question, suggesting that conventional astrophysics may have given rise to the objects we observe at all cosmic times.
To put it all together: yes, our Universe is not perfectly homogeneous and smooth, but instead is indeed lumpy and clumpy. It was born with small imperfections and inhomogeneities in it, and over time, those imperfections grew into the vast cosmic web, with galaxies, stars, planets, white dwarfs, neutron stars, and black holes all throughout it. Some regions really are of enormous density; others really are of a very low density.
But the Universe is not so lumpy or clumpy that our foundational assumptions about it — that it's isotropic and homogeneous on the largest scales — should be thrown out. The evidence for these properties of the Universe is very strong, as is evidence for the Universe being the same age and having (roughly) the same observed expansion rate in all directions and at all locations, save for the "evolution" that comes along with one simple fact: looking far away in space implies looking farther back in time.
I expect timescape cosmology to remain an area of interest for a few select researchers, but not to gain a broader following based on this research. It's exciting that a cosmological test has been concocted, but the truth is that dark energy's existence is now based on a wide, robust suite of evidence that's so comprehensive that even if we ignored all of the type Ia supernova data entirely, we would still be compelled to conclude that dark energy exists. It's important to keep your mind open to new ideas, but to always let reality itself rein you back in. Like many new ideas, the timescape cosmology simply withers when faced with the full suite of cosmological evidence.
Send in your Ask Ethan questions to startswithabang at gmail dot com!
Sign up for the Starts With a Bang newsletter
Travel the universe with Dr. Ethan Siegel as he answers the biggest questions of all
No comments:
Post a Comment